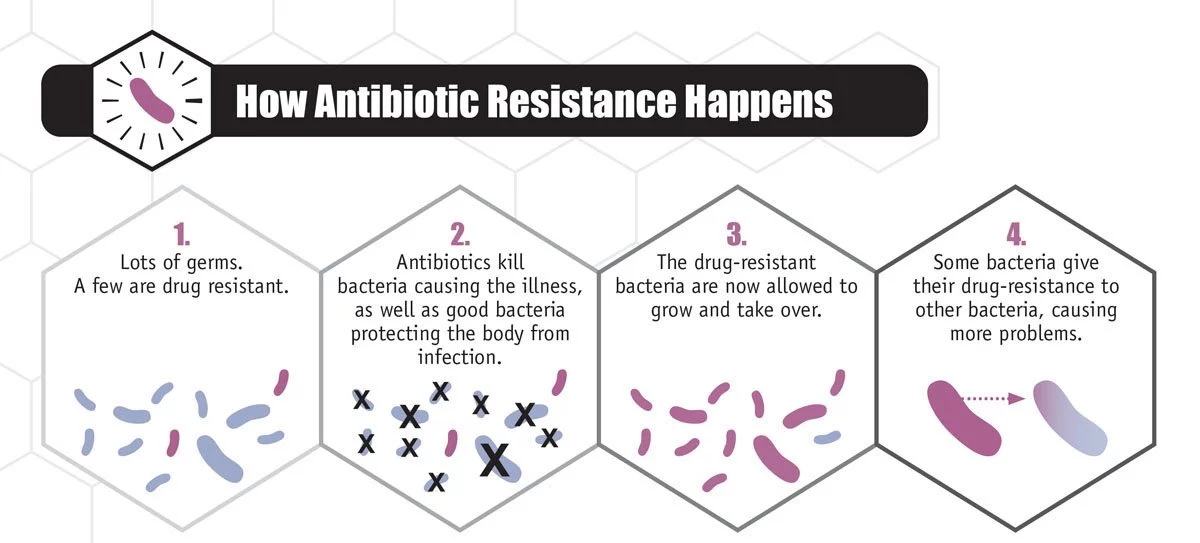
Science can border on science fiction. Designing swarms of infinitesimal semiconductors that, shined with green light, turn standard oxygen into a superoxide that makes life hell for drug-resistant bacteria – that qualifies.
A diverse University of Colorado team led by Anushree Chatterjee, PhD, did just that. Chatterjee, an assistant professor of chemical and biological engineering at CU Boulder, teamed with colleague Prashant Nagpal, PhD, to design and create these quantum-dot semiconductors and establish that they worked. To test them, they sought some of the toughest, nastiest microorganisms they could get their hands on. They turned to Nancy Madinger, MD, at UCHealth University of Colorado Hospital (UCH).
Madinger, an infectious disease specialist and medical director of UCH’s Clinical Microbiology Laboratory, opened a freezer set to minus-106-degrees Fahrenheit one recent morning. Inside frosty boxes, the Microbiology Lab stores about 15,000 samples of what she refers to simply as “organisms” collected over the past two decades. The collection’s main purpose is day-to-day infection control and patient care – her lab continually runs tests that, among many other things, help physician colleagues identify what’s causing an infection and what drug or drugs might best stop it. Then they freeze and store the samples. Because UCH treats many seriously ill, immunocompromised patients, it’s a trove from the perspective of those tracking and fighting antibiotic resistance.
Antibiotic resistance
“Antibiotics came into general use after World War II. For about 50 years, we were winning,” Madinger said. “But for the past 20 years, we’ve been losing. Unfortunately, that’s been accelerating, and in the past five years, it’s become quite dire.”
Michelle Barron, MD, medical director for infectious disease at UCH, put the antibiotic resistance problem in the really long-term context.
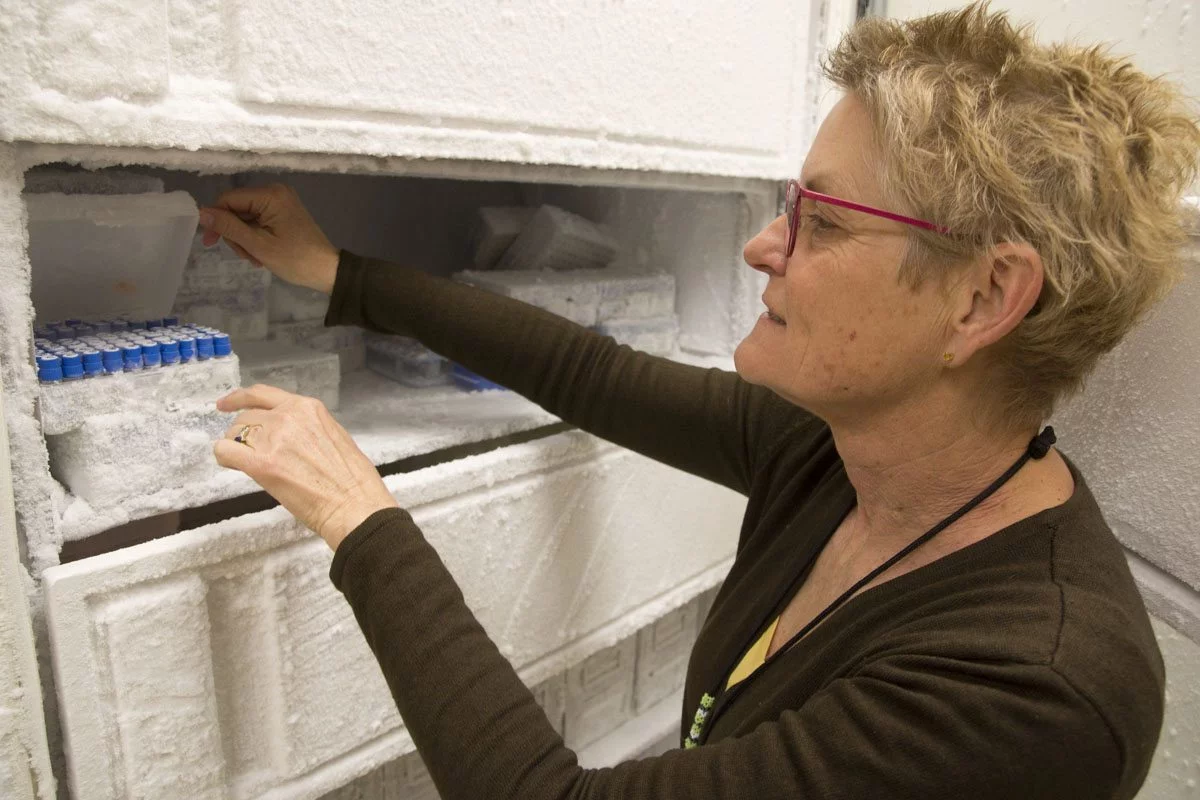
“Bacteria have been here how many millions of years compared to humans? And how did they survive all these crazy temperatures and dinosaurs and asteroids? They adapted,” Barron said. “Drug resistance is just another adaptation.”
Madinger said she used to came across infections that were “very hard to treat,” but could usually find a drug in the pipeline to handle it.
“And now I can’t,” she said.
She’s not just sitting back and lamenting that fact. In addition to her work with Chatterjee, she’s submitting organisms to six studies aimed at tracking antibiotic resistance on a national scale, Madinger said. In addition, the pharmaceutical companies tap into growing collection to test new drugs, she added.
Fearsome five
The organisms Chatterjee wanted were the sorts of “superbugs” driving today’s epidemic of bacterial drug resistance, one infecting an estimated 2 million people and killing 23,000 each year in the United States, and at a cost of about $20 billion in direct health care expenses alone. The worst of these are multi-drug-resistant bacteria, which, true to their name, have evolved to shrug off assaults from one antibiotic after another.
Madinger and Chatterjee settled on a microbial rogue’s gallery of a few dozen strains from the deep-freeze, which Chatterjee’s team DNA-sequenced and reduced to a handful, including methicillin-resistant Staphylococcus aureus (MRSA); Klebisella pneumoniae; Salmonella typhimurium; and two E. coli strains, one of which was resistant to no fewer than nine antibiotics.
Chatterjee’s idea was sparked by previous science that showed bacteria under antibiotic assault to have slightly higher volumes of reactive oxygen species, which are molecules that oxidize other molecules by robbing them of electrons. The same thing happens when oxygen in the air causes metal to rust.
“So we thought, ‘How about we engineer antibiotics that create a controlled amount of reactive oxygen species?’” Chatterjee said.
Go small
To do that, she enlisted Nagpal, a nanomaterials expert, who came up with cadmium-telluride semiconductors just two to four nanometers (billionths of a meter) across – small enough to penetrate bacterial cell walls. They would stay inert unless exposed to a particular green light (one with a wavelength of about 520 nanometers). The light would awaken nanoparticles as if someone had opened the blinds on a bright morning, causing them to kick off electrons in a sort of protest. Those electrons would be of an energy precisely calculated to transform the oxygen floating in and around the nanoparticle-infused bacteria into a superoxide bath, which Chatterjee and Nagpal figured would kill bacteria.
In a Nature Materials report published in January 2016, they showed that it worked. In a follow-up Science Advances paper published this month, they combined the nanoparticles with antibiotics the bacteria were otherwise resistant to. They found that the nanoparticles paved the way for the antibiotics to work far better than they otherwise would have.
The nanoparticles work as an antibiotic supercharger, Chatterjee said, because the bacteria must divert resources to overcome the superoxide stress broadly imposed on their biological workings. That makes them more susceptible to antibiotics. Traditional antibiotics, in contrast, rely on small molecules that, for example, might try to blow holes in a bacteria’s cell wall.
“Conventional small molecules target proteins,” Chatterjee said. “And here we target a process.”
Need to evolve
Because that process involves many of the systems that make bacteria tick, that process should be harder for them to overcome than the targeted assaults of small molecules, Madinger said.
“We will always be working with a moving target because bacteria are very clever,” she said. “If we develop something that targets something on their cell wall, they’ll figure out a way to modify their cell wall and survive.”
These semiconducting-nanoparticle-induced superoxides, though, exploit the fact that bacteria are killed by reactive oxygen species – “it’s taking advantage of a lethal foe that they already have,” as Madinger put it.
Given the need for exposing the nanoparticles to light, the approach shows the most promise for infections at or near the surface of the skin – in particular, those associated with wounds or burns. There’s certainly many who could benefit, said Laura Madsen, RN, burn outreach coordinator for the Burn Center at UCH.
“Infection is still the major thing that kills burn patients,” she said.
Chatterjee said the next step is to move forward with larger animal models (so far, they’ve gone as far as flatworms), and she hopes to further partner with physician faculty to speed up the process of moving her work from the brink of science fiction to patients and UCHealth and beyond.
“We should be evolving in a way that can keep up with evolving pathogens,” she said.
Which is to say: very, very quickly.